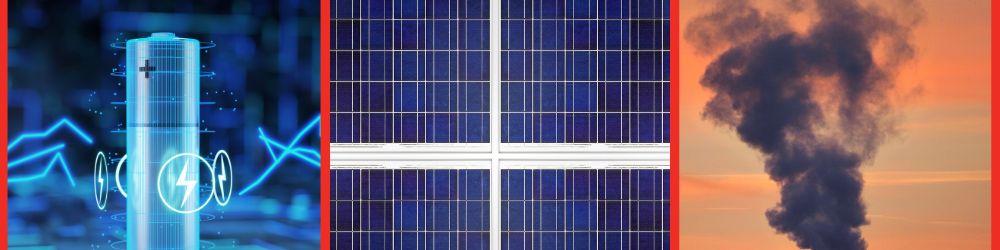
Microspectroscopy of Nanostructured Arrays
Nanostructured arrays are transforming how we approach challenges in energy, healthcare, and advanced optics. Their remarkable properties, such as tunable optical characteristics and enhanced surface areas, make them essential in cutting-edge technologies like solar cells and biosensors. To unlock their full potential, researchers need tools capable of analyzing their intricate structures and behaviors. Microspectroscopy, a powerful fusion of microscopy and spectroscopy, provides the precision and insights needed to characterize and optimize nanostructured arrays for advanced applications.
What Are Nanostructured Arrays?
Nanostructured arrays consist of nanoscale components like nanoparticles, nanowires, or nanotubes, systematically organized into defined patterns. These arrays exhibit remarkable properties:
-
Enhanced Surface Area: Promotes reactions in catalysis and improves electrode performance in energy storage.
-
Adjustable Properties: Allows the fine-tuning of optical, mechanical, and electrical behaviors.
-
Periodic Arrangements: Amplifies phenomena such as light diffraction and electrical conductivity.
Fabrication techniques include top-down approaches like electron-beam lithography and etching, as well as bottom-up methods such as chemical vapor deposition or nanoparticle self-assembly. The resulting materials have enabled innovations in photonic crystals, biosensors, and advanced photovoltaic systems.
The Role of Microspectroscopy
Microspectroscopy is pivotal in bridging the gap between the nanoscale structure of these arrays and their macroscopic performance. By combining the spatial precision of microscopy with the analytical depth of spectroscopy, it uncovers the localized properties of nanostructured arrays that govern their behavior and effectiveness in applications.
Key Techniques and Their Applications
1. UV-Vis-NIR Microspectroscopy
Measures how nanostructured arrays absorb, transmit, or reflect light across a broad spectral range.
Applications:
- Assessing optical resonances in plasmonic nanostructures.
- Mapping light absorption efficiencies in nanostructured solar cells.
- Characterizing optical effects of nanostructured arrays
- Understanding the physics of nanostructured arrays and characterizing how changes in the array density and shapes change the optical responses.
Advantages
This technique excels at accurately measuring thin film thickness, which is crucial for optimizing efficiency in applications such as solar panels, photonic devices, and anti-reflective coatings.
2. Raman Microspectroscopy
Detects vibrational modes in molecules, making it an ideal tool for analyzing chemical composition, structural uniformity, and stress distributions within nanostructured arrays.
Applications:
- Probing the crystalline quality of nanowires and nanotubes.
- Mapping stress patterns in photonic crystals.
Advantages
Raman microspectroscopy provides non-destructive and highly specific chemical characterization, enabling the precise analysis of molecular structures and material properties without altering the sample. This type of microspectroscopy is also effective for identifying strain, defects, and phase transitions in complex nanostructures.
3. Photoluminescence (PL) Spectroscopy
Studies light emitted by materials upon excitation, revealing key electronic properties like bandgaps and defect states.
Applications:
- Characterizing semiconductors in photovoltaic arrays.
- Identifying defects that may impede charge transport in energy storage devices.
Advantages
PL spectroscopy is highly effective for evaluating materials like quantum dots and nanocrystals. This is because it offers non-destructive analysis while pinpointing electronic properties critical for device performance.
4. Linear Polarization Microspectroscopy
Combines UV-Visible-NIR polarization spectroscopy with microspectroscopy to achieve unique optical effects. By measuring changes in the polarization state of light as it interacts with these arrays, this method provides insights into their structural, electronic, and optical properties. The technique is sensitive to anisotropies and plasmonic resonances, which are influenced by the size, shape, arrangement, and material composition of the nanostructures.
Applications:
- Providing the detailed studies of optical phenomena of nanostructured arrays
- Improving the performance of nanostructure array-based sensors.
Advantages
Polarization microspectroscopy is a valuable tool for applications in sensing, photonics, and the study of nanoscale optical phenomena.
5. Circular Dichroism Microspectroscopy
Circular dichroism microspectroscopy of nanostructured arrays is a technique that measures the differential absorption of left- and right-circularly polarized light, revealing chiral optical properties of the structures. Nanostructured arrays, especially those with intrinsic or induced chirality, exhibit unique CD signals due to interactions between light and their asymmetric geometries or plasmonic modes. This method is highly sensitive to the handedness, symmetry, and spatial arrangement of the nanostructures, making it valuable for studying chiral plasmonic effects, enantioselective sensing, and the design of advanced metamaterials for optical applications.
Applications:
- Studying the chiral properties of nanostructures.
- New tool to examine self-organized hierarchical chirality.
Advantages
Circular dichroism enables the study of light-matter interactions at an unparalleled level of detail. This makes it essential for designing advanced optical components like waveguides and photonic crystals.
Applications of Microspectroscopy in Nanostructured Arrays
Energy
Microspectroscopy allows for the precise mapping of charge transport and light absorption in nanostructured solar cells, improving efficiency. For batteries, microspectroscopy provides insights into electrode performance, enabling better energy storage and discharge rates.
Biomedicine
Functionalized nanostructured arrays play a key role in biosensing and drug delivery. Microspectroscopy helps map molecular binding sites and detect minute biochemical changes, leading to enhanced diagnostic tools and therapeutic systems.
Optics and Electronics
In photonics, microspectroscopy enables the study of optical resonances and defect states in photonic crystals and plasmonic arrays, driving the development of next-generation LEDs, sensors, and waveguides.
Environmental Science
Nanostructured catalysts designed for pollutant breakdown or water purification are optimized through microspectroscopy, which reveals reaction intermediates and hotspots critical for performance improvements.
Explore The Capabilities Of Microspectroscopy With Products From CRAIC Technologies
Microspectroscopy has become an indispensable tool for advancing nanostructured arrays, providing the precision and depth needed to understand their intricate properties. By enabling researchers to explore the optical, chemical, and electronic characteristics of these materials at the nanoscale, microspectroscopy fosters innovation in energy, biomedicine, and environmental science.
We, CRAIC Technologies, offer powerful solutions tailored to these challenges. Our UV-Vis-NIR microspectrophotometers are ideal for characterizing light absorption, reflection, and transmission, and so are key to optimizing solar cells and photonic devices. Complementing this, our Raman microspectrometers, linear polarization and circular dichroism capabilities also provide detailed molecular and structural insights, essential for identifying defects and improving material performance. Together, these tools equip researchers with the capability to unlock the full potential of nanostructured arrays.
With our advanced microspectroscopy systems, you can drive discoveries and accelerate innovations. Take a look at our website to learn more about how these tools can elevate your research.